Teaching Speed - Applying Force Quickly By Micheal Coonen Originally Published in Techniques Magazine - Official Publication of the USTFCCCA
A study performed by Weyand, Sternlight, Bellizzi and Wright at Harvard University in 2000 looked at what the determining factors were for a greater top speed in active men and women between the ages of 18 and 36. Participants were asked to perform a multitude of tests that measured their stride length, stride frequency, turnover speed, ground contact time, ground contact force and top speed. The study found that at top speed there was no significant difference in turnover time between the fastest of runners and the slowest of runners, making turnover a non-influential variable. It was shown, however, that stride length increased in all runners when they increased their running speed. It was also determined that the variables that had the greatest difference from slow runners to fast runners were ground contact time (GC1') and ground contact force (GCF). The ground contact force generated by the fastest runner was almost 1.26 times greater than that generated by the slowest runner. The slowest runners were discovered to spend almost twice as much time on the ground than the fastest runners. Weyand, Sternlight, Relli7zi and Wright showed that shorter ground contact time plus greater ground contact force was the new formula for speed. Yet another study by Chang and Kram completed in 2007 examined the reduced speeds of running on a curved track compared to a straight track and explored the limiting factors on the maximum speed of curved track running. Using a model created by Greene (1985), Chang and Kram explained that ground contact force on a curved track is a combination of vertical GCF and lateral GCF. This results in a decrease of vertical GCF leading to increased ground contact time. As discussed earlier; longer ground contact times cause a decrease in maximal speed. This study went on to find that when sprinting on a curved track the inside leg did not produce as much GCF as the outside leg, nor did it produce as much GCF compared to straight track running. The reduced GCF from the inside leg and resulting increase in ground contact time while sprinting on a curve led to lower maximum sprint speeds. In some cases, 200-meter sprinters were 0.4 seconds slower while running on a curved track compared to a straight track (Chang and Kram, 2007). The referenced studies indicate that increasing maximal sprinting speed is accomplished by increasing the amount of force applied to the ground and decreasing the amount of time each foot strike spends on the ground. Some may argue that there are other or more important factors that determine top speed sprinting. Turnover, stride length and knee drive are three that are often discussed (Murgia, 2008). As we know from the previous research, turnover remains a constant in all sprinters, thus eliminating it from the discussion (Weyand et al., 2000). I will further examine the concepts of stride length and knee drive and how they affect maximal speed sprinting. THE POTENTIAL Stride length can be defined as the distance an athlete moves down the running surface between the toe-off of each foot strike. The further down the track a sprinter can relocate their body with every stride, the faster and more efficient that sprinter will be. Current 100-meter and 200-meter world record holder, Usain Bolt, only requires 40-41 strides to complete a 100-meter dash compared to the 45-46 strides it took U.S. greats Maurice Greene and Tyson Gay. That means Bolt's stride is on average 0.22 meters greater than that of Greene and Gay (Shinabargar, Hellrich and Baker, 2010). But what causes an increase in stride length? Ground contact force. The more force a sprinter applies to the ground the further it will propel them down the track (Bushnell and Hunter, 2007). This increase in stride length (hip displacement) will elicit the stretch- shortening response of the hip flexors allowing for a greater knee drive. Often times, in an attempt to increase stride length, sprinters will overstride to compensate for a lack of ground contact force. When an athlete overstrides, or reaches with their stride, it places unnecessary strain on that athlete's hamstrings, creates breaking forces and reduces the plyometric effect of the gastrocnemius-soleus complex which is vital in maximum speed sprinting. An overextended stride length also creates horizontal breaking forces which results in a loss of speed (Nummela, Keranen and Mikkelsson, 2007). Stride length should be limited by technique. Do not allow an athlete to reach in front of them with their foot. Rather place an emphasis on displacing their hips further down the running surface with every stride. This will ensure that their foot strike remains underneath the hips, where optimal forces can be generated by the lower extremity musculature and proper sprint mechanics are maintained (Bushnell and Hunter, 2007). KNEE DRIVE Knee drive is regulated by the stretch-shortening response of the hip flexors, or simply put; knee drive is a plyometric activity. During a stretch, the elastic fibers within the muscle tissue reach a point where they recoil and cause the crossed joint to displace their hips; a greater stretch will be put on the hip flexor. This stretch will elicit the stretch-shortening response of the hip flexor causing a fast and passive flexion of the hip joint. According the USA Track and Field Level 1 Coaching Curriculum (2006), knee drive has a direct relationship to the amount of force applied to the ground. A low knee drive is a result of weak ground contact force. The inability to produce adequate knee drive during the latter portions of a 200-meter or 400-meter race is a result of decreased ground contact forces during those last few meters. Greater knee drive and an increased stride length are byproducts caused by a greater force being applied to the running surface. By understanding that maximum speed is a product of greater ground contact forces and shorter ground contact times there are many ways you can introduce those concepts into your training. The following are just a few of numerous ways that these ideas can be effectively carried over and applied when training sprinters. SPRINT DRILLS Special attention should be paid to the execution of sprint drills during warm-ups and practice. There should always be an emphasis placed on proper sprint mechanics, i.e. dorsiflexion of the ankle, upright posture, neutral pelvic alignment, limiting upper body rotation, etc. (Thompson, Bezodis and Jones, 2009). In addition to correct sprint mechanics additional emphasis should be put on applying force to the ground during these drills. Instead of allowing an athlete to place their foot down while performing drills such as A-skips and A-runs, they should be taught to put their foot down with force. Athletes are often too focused on lifting their knees high. As previously discussed, we know knee lift is generated by the stretch of the hip flexors and the force applied to the ground, not the active recovery of the knee. The more force an athlete applies to the ground, the more the knee recovery will passively take place. With this in mind, more time should be spent placing an emphasis on the application of ground contact force. Cues that work well during the supervision of sprint drills are "Put your foot down. Meet the ground; don't let the ground meet you. Attack the ground. Be aggressive." Force application can also be worked in your strength and conditioning program. Complex training is yet another way to influence ground contact force. Complex training is the combination of a heavily resisted exercise performed at comparatively slower speeds paired with a lightly resisted exercise performed at comparatively faster speeds (Chiu et al., 2003; Hodgson, Docherty and Robbins, 2005). Typically you will find examples of complex training as performing a set of an Olympic lift (ex: power clean or hang snatch) superset with a set of a body weight exercise (ex: box jump or split jump). The importance of complex training is in the order of the lifts. It is crucial the heavily resisted lift is performed prior to the lightly resisted lift. By asking the body to move heavier weight it creates a level of excitement in the motor neurons of the muscle allowing for greater recruitment of those motor neurons in subsequent sets. This is called post-activation potentiation (Chiu et al., 2003; Hodgson et al., 2005). It is like lifting a large, empty box when you believe it is full and heavy. The box will fly through the air because you used greater force than what was needed for that activity. In your training, if you perform a heavy, yet explosive Olympic lift prior to a lightly resisted exercise, the body will recruit more motor neurons than what was required resulting in a much more explosive lift. Overtime adaptation will occur and the body should continue to recruit those motor neurons in lightly resisted exercises, i.e. box jumps, block starts or running (Hodgson et al., 2005). Attempt to incorporate complex training into your strength and conditioning plan at least two days a week This theory can also be worked into your training on the track By performing resisted explosive lifts prior to block starts or top speed work it will elicit the same response seen in the weight room. PLYOMETRICS Plyometric exercises are a great way to train athletes to minimize the amount of time spent on the ground. A plyometric exercise is defined as a rapid stretch and subsequent contraction of the employed muscle groups that requires limited time for force development (less than 0.2s).To begin; incorporate movements that require the athlete to perform the exercise quickly over a short obstacle. Limiting the obstacle to no more than 16 inches in height ensures that the primary force used to clear the obstacle is a true plyometric response rather than an active contraction (Markovic and Mikulic, 2010). The primary focus of any true plyometric exercise is to encourage the minimizing of the ground contact time. Taller obstacles require the athlete to spend more time on the ground generating the forces necessary to clear that obstacle. As time progresses and adaptations occur, obstacles can be increased in height, as long as ground contact time remains at a minimum (Ebben, Fauth, Garceau and Petushek, 2011). An example of a plyometric progression is beginning with simple line drills. Instruct the athlete to "jump" over a line on the floor in a forward-backward motion, while trying to spend as little time as possible. Once time for adaptation has been allowed, move to clearing shorter obstacles such as a 6-inch hurdle. In mature or elite athletes the overload principle may be applied by increasing the height of the object to be cleared. Strong evidence has been found that as long as the athlete performs the rep with the intent to be as explosive and as fast as possible, that velocity and power specific adaptations occur in the nervous system (Behm and Sale, 1993; Khlifa, Aouadi, Hermassi, Chelly, Did, Hbacha and Castagna, 2010). Overloading can be harmful to younger developing athletes, but in more mature competitors this can be a great way to increase power. Special attention should be paid to the amount of touches performed while completing plyometric exercises. The touches should be limited to ensure the athlete remains free of injury. Keep in mind that sprinting alone is classified as a plyometric activity, so a large increase in such activities can lead to an increase in the risk for injury. BOUNDING Bounding is a branch off of plyometrics and an excellent way to incorporate the stretch-shortening cycle, force application and hip displacement in one training exercise. Bounding provides specificity in sprint training by focusing on both vertical and horizontal ground contact forces while limiting ground contact time. The objective of bounding exercises is to increase the amount of force an athlete applies to the ground and distance in which their hips are displaced down the running surface while decreasing the amount of time it takes to generate that force. Bounding has been shown to decrease ground contact time and improve sprint performance up to 40 meters (Rimmer and Sleivert, 2000). Begin bounding training with shorter, slower movements. Bounding from hash marks to hash mark on a football field from a standing start is a great place to begin. Slowly progress to bounding further distances and allow for a short run-up to increase speed. Sprint drills, complex training, plyometrics and bounding are just four ways you can attempt to increase the forces your sprinters place on the track and limit the amount of time it takes them to do so. As a coach, you can be creative in your attempts to decrease ground contact time and increase ground contact force. Keep in mind that every exercise or drill performed should have a purpose and relate in some way to your ultimate goal, creating faster sprinters. Know the reasons behind performing every drill and make sure they are executed in a manner that benefits maximum speed development. REFERENCES Ackland, T, Elliot B., & Bloomfield, J. (Eds.). (2009). Applied Anatomy and Biomechanics in Sport. Champaign, IL: Human Kinetics. Behm, D. & Sale, D. (1993). Intended rather than actual move¬ment velocity determines velocity-specific training response. Journal of Applied Physiology.74, 359-368. Bushnell, T, & Hunter, I. (2007). Differences in Techniques between Sprinters and Distance Runners at Equal and Maximal Speeds. Sports Biomechanics, 6(3), 261-268. doi: 10.1080114763140701489728 Chang Y.H., & Kram, R. (2007). Limitations to maximum running speed on flat curves. The Journal of Experimental Biology. 210,971-982. doi: 10.12421jeb.02728 Chiu, L., Fly, A, Weiss, L., Schilling, B., Brown, L., & Smith, S. (2003). Postactivation potentiation response in athletic and recreationally trained individuals. Journal of Strength and Conditioning Research. 17(4), 671-677. Ebben, W, Fauth, M, Garceau, L. & Petushek, E. (2011). Kinetic quantification of plyometric exercise intensity. Journal of Strength & Conditionsing Research. 25(12), 3288-3298. Greene, P.R. (1985). Running on flat turns: Experiments, theory and applications. J. Biomechanical Engineering. 107, 96-103. Hodgson, M, Docherty, D., & Robbins, D. (2005). Post-activation potentiation underlying physiology and implications for motor perfor-mance. Sports Medicine, 25(7), 385-395. Khlifa, R., Aouadi, R., Hermassi, S., Chelly, M, Jlid, M, Hbacha, H, & Castagna, C. (2010). Effects of a plyometric training program with and without added load on jumping ability it basketball players. Journal of Strength & Conditioning Research. 24,2955-2961. Markovic, G. & Mikulic, P. (2010). Neuro¬musculoskeletal and performance adaptations to lower extremity plyometric training. Sports Medicine, 40(10), 859-895. Murgia, C. (2008). SeparatingFact from Fiction: Increasing Running Speed. The Journal of Physical Education, Recreation & Dance, 79(8), 17-19 Nummela, A A., Keranen, T. T, & Mikkelsson, L. 0. (2007). Factors Related to Top Running Speed and Economy. International Journal of Sports Medicine, 28(8), 655-661. doi:10.10551s-2007-964896 Thompson, A., Bezodis, L, & Jones, R. (2009). An In-depth Assessment of Expert Sprint Coaches' Technical Knowledge. Journal of Sport Sciences, 27(8), 855-861. doi: 10.1080102640410902895476 Rimmer, E. & Sleivert, G. (2000). Effects of a plymetrics inter¬vention program on sprint performance. Journal of Strength and Conditioning Research, 14,295-301. Shinabargar, A.J., Hellrich, M, & Baker, B. (2010) What makes Usain Bolt unique as a sprinter? The physics teacher. 48,365. doi: 10.111911.3479707 USA Track & Field. (2006). Coaching education: Level 1 curricu¬lum. The sprint events, 55-62. Weyand, P., Sternlight, D., Bellizzi, M, & Wright, S. (2000). Faster top running speeds are achieved with greater ground forces not more rapid leg movements. Journal of Applied Physiology, 89, 1991-1999. BIO Michael Coonen is the Sprints, Hurdles and Relays coach at St. Cloud State University.
|
|
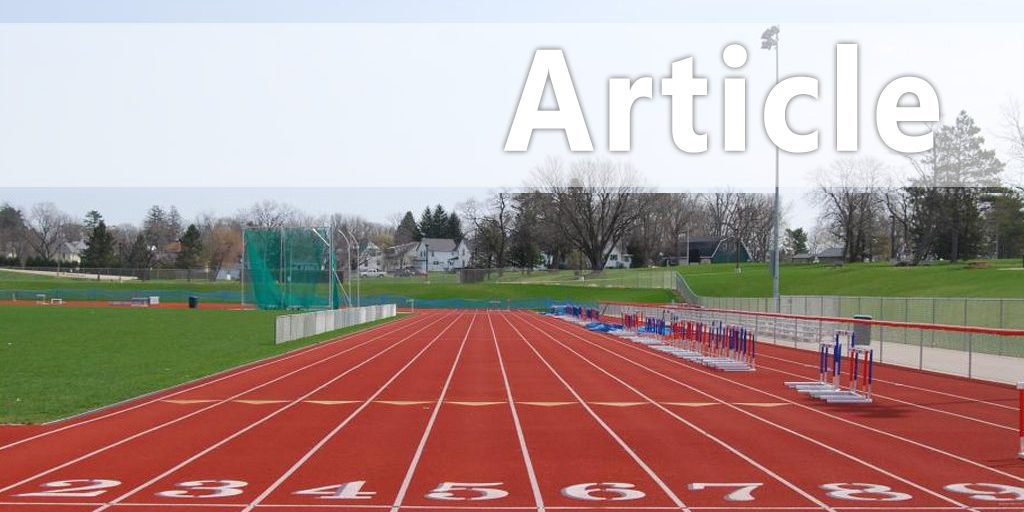