By: Andreas V. Maheras, Ph.D. Originally Published in: Techniques Magazine Provided by: USTFCCCA The javelin thrower and their implement can be considered as a system moving in space and time, with a proper synergy between the two resulting in the maximum distance thrown. The javelin thrower them self can also be considered as a system - a system which is made of a number of body parts having their own mass and their own mass distribution, with the neuromuscular system being in charge of controlling the interaction of those individual parts. From a dynamic point of view, the final product of that interaction is the result of another interaction between muscular forces and external forces - the latter being the inertia of the implement and the body parts and also the force of gravity. General knowledge about movement interaction and coordination is essential for maximizing the positive mechanic effect on the throw. LEG DYNAMICS A well-known axiom of all the throwing events states that effective throwing relies heavily on the contribution of the legs. In javelin throwing, a few factors that are crucial for optimal performance include controlling the velocity of the system in the various phases of the throw, the position(s) of the body and that of the javelin, the acceleration path during the delivery of the implement, and control of the javelin at the moment of the final effort. Back leg. Hypothesizing that there is a need for high system speed before delivery, the back leg's action (along with that of the upper body) should ensure minimal deceleration of the system's velocity during the final stride. Decreases in the speed of the body may range between 0.31 to 0.67 m/sec. (Bartonientz, 2005). A sound technique should be geared towards reducing deceleration and "back lean" at the moment the right foot lands, following the execution of the impulse step. In turn, the movements preceding the impulse step will affect the actual position of the upper body at the moment the right foot touches down for the final stride. A high drive of the right leg during the impulse stride will most probably lead to a pronounced, backwards leaning upper body (figure 1). The explanation for this lies with Newton's third law (action-reaction) while the thrower is airborne. A better alternative may be one where, during the impulse, the left foot actively grabs the ground while the right leg is executing a shorter duration, scissors-like impulse. The idea of a backward lean of the torso may still be a misunderstood concept. It was considered that such a lean would enable the thrower to increase the path of force exertion on the implement. However, for most throwers, the greater the backward tilt of the torso at the moment of landing on the right foot, the greater the loss of the system's velocity as previously implied. In addition, a reduced back lean will also contribute to a higher carry of the javelin (figure 1), which has shown to be important in improving the aerodynamic position of the javelin at the time of release (Leigh et al., 2010; Best et al. 1993; Best et. al., 1994). Although several years ago the thought of increasing the velocity of the system - or even keeping it constant - after the execution of the impulse step was considered unattainable, the possibility of that occurring may still exist. As the thrower executes an active impulse with the left foot, she keeps the upper body in a neutral and a rather vertical position. Following that impulse and during the forward "hovering" phase, the center of mass (c.m) is moving pretty much horizontally, with no appreciable upward or downward movement. The thrower's big goal at this time is to maintain her horizontal velocity while keeping the c.m. unchanged. However, there is a big danger of slowing down upon landing on the right foot. Two actions that may prevent that from happening are a) the actual landing to occur exclusively on the toes of the right foot and b) with a backwards pulling or "pawing" action on the part of the right foot as it is about to land. Therefore the javelin thrower thinks of pulling backward with her right foot, as if to increase horizontal speed. She normally may not succeed at increasing horizontal speed, but by thinking of pulling back with the right foot and of gaining horizontal speed, she will actually succeed at maintaining horizontal speed, which was a minimal original goal (Dapena, 2019). Following those two actions, it is going to be hard for the thrower to continue pushing backward with the right foot for much longer after the left foot plants. This is because, ideally, the right leg will already be in such a backward position by then. One cannot push back any more because the right leg is at or close to the limit of its backward range of motion. As for the significance of the maintenance of high system speed during the final strides, analysis data of velocities of the center of mass for a full approach show that top athletes exhibit higher body velocity at the beginning of the delivery phase and that they also achieve the longest throws when their run up speed is at its highest. Generally, the higher speed levels and longer impulse strides lead to longer distances thrown (Leigh et al., 2010). Regarding the actual position of the right foot, after its final landing, in relation to the direction of the throw, that varies between almost zero degrees, i.e., in line with the direction of the throw, and ninety degrees, i.e., perpendicular to the direction of the throw. The first method may reduce the influence of a right leg drive (if any), whereas the latter may lead to knee injury caused by an inward bending. Therefore a right foot position somewhere in between, at forty five degrees, may be the preferred right foot landing position for many throwers (Sing, 1984). Front Leg. The action of that leg is power demanding, as it provides the means by which the thrower will abruptly stop her forward movement and initiate the throwing action. Both from a mechanical and a physiological point of view, a generously flexed front leg will not properly create the conditions for the thrower to achieve the desired "arch" position. Depending on the instance of the delivery phase, any observed knee flexion throughout this phase will vary a few degrees, with 180 degrees being a straight leg position. Smaller left knee flexion values (and high knee stability) are associated with higher performances (Mahmoud, 2010; Moriss et al., 1997). The front leg should be planted as fast as possible while the thrower should strive to limit any constraints in regards to the velocity of the system, the position of the front leg at the moment the back leg touches down, along with the action of the right foot itself. Time values as low as 0.14 sec. have been recorded (Morris et al., 1997) between right foot and left foot landing during the throwing stride. A firm front leg will allow for a short translation of the of the c.m during the final effort. Experimental data (e.g., Morriss et al., 1997) have shown the "firmness" of the front leg to be a reliable criterion for performance improvement. Another factor determining the effectiveness of the front leg is the length of the throwing stride itself. High caliber throwers tend to employ a longer throwing stride, whereas throwers of lesser abilities employ shorter strides which are also characterized by steeper ground reaction forces. From a mechanical point of view, as the front leg touches down, the ground force which is created at that moment passes through the c.m and in turn results in the creation of a counterclockwise angular momentum of the upper body. At this moment, the direction of the force is at a high angle (see figure 3). This provides the foundation for the creation of the desired muscular tension and the "arch" position, which is created by the combination of an inert shoulder and arm, coupled with the aforementioned angular momentum. As the movement continues, the ground force will quickly change its direction and size. As far as the direction, that will act along the front leg, whereas the size of it will reach its maximum. The direction of the angular momentum changes to clockwise (figure 2) and as it occurs, it further enhances the thrusting of both the chest and the throwing arm, culminating with the projection of the javelin. This way there is a transfer of angular momentum from the body to the throwing arm (LeBlank & Dapena, 1998). The angular momentum and its direction will affect the performance outcome particularly as it relates to the influence the front leg has upon it. As that leg flexes, there is a reduction in the size of the ground reaction force as well as a change in its direction. There is an observed strong dependence between kinematic parameters (like joint angles and velocities), and performance levels - so much so that it is thought that differences in the former can explain differences in the latter. From a technical point of view, those differences manifest themselves as, a) a smaller size ground reaction forces at right foot touch down after the impulse step, b) a more dynamic landing of that same foot with less backwards tilting of the torso, c) a maintenance or even increase of the system's velocity due to that dynamic activity of the back leg, d) a very dynamic activity of the front leg which generates high ground reaction forces and results in a rapid deceleration of the system and a dramatic energy transfer from the body to the javelin (Bartonietz, 2005). The high demand placed on the front leg may limit the number of throws which can be attained during practice. In top athletes, the greatest amount of deceleration occurs during the first third of the delivery phase as revealed by ground reaction force data (figure 4). A controlled throw in practice with a relatively low body speed of say 4m/sec., may eventually decelerate to 2 m/sec. in about 0.14 seconds as the left foot plants. This change in energy is approximately three and a half times smaller than that experienced in competition (Bartonietz, 2005). Therefore, as the run up speed increases during competition throwing, the energy demands also increase. On the other hand, the time to transfer the energy remains virtually constant, and this way the overall power required on the part of the thrower is fourfold because of the square influence of the velocity of the body on the energy and, in turn, on the power. From a mechanical point of view, this explains why less powerful athletes, who demonstrate an acceptable throwing pattern during practice and at lower speeds, fail to do the same during a full approach. There is simply not adequate amount of power (Bartonietz, 2005). UPPER BODY AND THROWING ARM The momentum transfer theory is used to explain the velocities of the various body segments over time and expresses the impulse transmission to the implement. The big picture in applying this theory for a javelin thrower is that initially the thrower+javelin system is accelerated to acquire momentum (mass x velocity) and subsequently, a deceleration occurs which first includes the legs and the lower parts of the torso, resulting in the acceleration of the upper parts of the torso. In turn, the upper parts of the torso also decelerate while the upper arm, forearm and hand, in that order, also accelerate ending up in a "snapping" projection of the javelin. The seamless execution of the sequence of the above described movements will result in a smooth, not forced throw, which is "effortless" and is the result of an effective use of momentum transfer. This transfer occurs from muscles situated nearer to the center of the body (proximal) to those situated away from the center of the body (distal), following an optimal delay in their activation, and based on the mechanical interaction between the proximal and distal muscles involved. On the other hand, that seamless execution does not depend on the transfer of momentum alone in order to occur. Although effortless, in terms of lack of unnecessary and disorderly muscular activity, the delivery does require intense and coordinated muscular activity. This implies the development of skills such as the ability to delay the action of the throwing arm (Bartlett et. al., 1996) and generally, develop a "feel" for the javelin. The delaying of the arm in particular has a significant impact for performance as it is central in developing the necessary pretension and stretching just before the javelin release. The maximum of the "bow" phase can be considered the moment in time when the upper arm starts rotating internally or the moment when that arm stops rotating externally and the final acceleration of the javelin begins. At that exact time, the potential of the stretch-shortening cycle is employed, and there is an optimal body speed at which this cycle would work most efficiently. It has been hypothesized that a great amount of the difference in distance thrown between low speed approaches and higher speed approaches is due to a compromised efficiency of the musculotendinous system to indeed utilize the stretch - shortening cycle in lower speed conditions. ENERGY FLOW CONSIDERATIONS During the final effort which follows the planting of the front leg, the traditional model of energy flow as described by Kreighbaum & Barthels (1981), argues about a proximal to distal energy flow, as described above, and it states that the distal limb, the forearm, will accelerate because the proximal limb, upper arm, will decelerate to ensure appropriate momentum transfer. On the other hand, Bartonietz (2005) argued that it is not the proximal limb that decelerates in order to accelerate the distal limb, but it is the other way around. Invoking Newton's action-reaction law, he stated that the proximal limb decelerates as a reaction to the acceleration action of the distal limb. Therefore it could be that distal limbs affect the proximal limbs more than the other way around (also in Kulig et al., 1983). This possibility may be essential regarding the overall final effort in javelin throwing and the movement of the upper body in particular. As the front leg plants, the velocity of the torso is virtually constant (figure 5), and will be influenced by the reaction of the arm acceleration. If the thrower, employing the proximal to distal order of energy flow, stops the forward movement of the trunk, in order to provide "proper" energy transfer to the arm, she will fail to work through with the upper body and instead, she will remain in a generally upright position, which will result in a decrease in release velocity, a high angle of release and a lack of a rolling in of the elbow. Therefore, an observation of the velocity changes of the various limbs may be sufficient in evaluating the individual throwing pattern of a thrower, but may not be sufficient in evaluating the contribution of the various body segments to the throwing movement itself. CONCLUSIONS The javelin thrower needs to be trained to take advantage of the dynamics of her body segments so she can generate the maximum power and energy. A few important technical points to consider avoiding include, a) a low speed run up, or a run up that leads to a passive instead of an accelerating penultimate stride, b) an inactivity of the right leg which is manifested with a marked loss of speed and a lack of pushing the right side forward, c) a planting of the front leg in a flexed position, d) a steep planting angle of the front leg, e) an untimely delivery, or f) a premature movement of the throwing arm with the ensuing deficit in muscular tension. The front leg in particular requires conditioning, both technical and muscular, to be able to bring the system to a quick stop. General and specific power can be developed by strength exercises and by practicing under approach speeds, that match those of competition. The amount of body speed lost during the delivery phase as a result of a firm block, can serve as a gauge of throwing efficiency, taking into account the initial speed. Because of the lower power demands, the lower the initial speed, the easier it is to rapidly decelerate, com-pared to achieving the same deceleration at higher initial body speeds. Generally, but not always, the lower the velocity of the center of gravity at release, the higher the velocity of release, an observation that accentuates the importance of the action of the front leg. REFERENCES Bartlett, R., Muller, E, Lindinger, S. Brunner, F., & Morriss, C. (1996). Three dimensional evaluation of the kinematic release parameters for javelin throwers of different skill levels. Journal of Applied Biomechanics, 12, 58-71 Bartonietz, K. (2005). Javelin throwing: An approach to performance development. In Biomechanics in Sport, ed. Zatsiorsky, V. pp. 401-434. Best, R., Barteltt, R., Morriss, C. (1993). A three dimensional analysis of javelin throwing technique. Journal of Sports Science, 11, 315-328. Dapena, J. (2019). Personal communication. Kreighbaum, E, & Barthels, K. (1981). Biomechanics: A Qualitative Approach for Studying Human Movement. Minneapolis, MN. Kulig, K., Nowacki, Z., Bober, T. (1983). Synchronization of partial impulses as a biomechanical principle. In: Biomechanics VIII-B. Proceedings of the 8th International Congress of Biomechanics. Leigh, S. Liy, H, & Yu, B. (2010). Associations between javelin throwing technique and aerodynamic distance. International Symposium on Biomechanics in Sports. Conference Proceedings Archives, Vol. 28. Leigh, S. Liy, H, & Yu, B. (2010). Associations between javelin throwing technique and release speed. International Symposium on Biomechanics in Sports. Conference Proceedings Archives, Vol. 28. Mahmud, E. (2010). Movement analysis for javelin throwers in the Quatar 2009 championships. International Symposium on Biomechanics in Sports. Conference Proceedings Archives. Morriss C., Bartlett, R., & Fouler, N. (1997). Biomechanical analysis of the men's javelin throw at the 1995 world championships in athletics. IAAF New Studies in Athletics, 12:2, 31-41. Morriss C., Bartlett, R. (1994). The Sing, R. (1984). The dynamics of the javelin throw. Reynolds publishers, Cherry Hill New Jersey DR. ANDREAS MAHERAS IS THE THROWS COACH AT FORT HAYS STATE UNIVERSITY IN KANSAS AND A FREQUENT CONTRIBUTOR TO THIS PUBLICATION. |
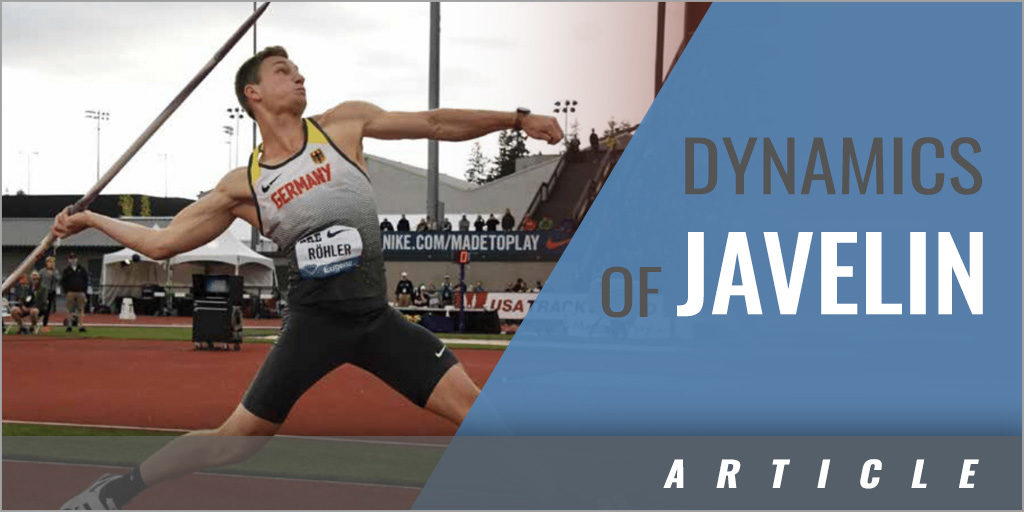