Body Composition - Methods and Importance for Performance and Health |
By: Donald R. Dengel, Ph.D A., Olivia H. Dengel
Originally Published in: Techniques Magazine Provided by: USTFCCCA Unlike some sports, track and field athletes come in a variety of shapes and sizes, as well as body composition. However, within a given track and field event the athletes competing in that discipline have very similar physiques and body compositions. Some events in track and field are considered gravitational in nature where total body mass restricts performance due to mechanical or gravitational reasons (e.g., high jumping, distance running, etc.) (Auckland et al., 2012). In these events, some athletes may resort to different methods to reduce or maintain a low body mass to gain a competitive advantage. However, having a low body mass does not always guarantee success in these events and in some cases an extremely low body mass may, in fact, be harmful. For example, an athlete may restrict their diet and not have adequate calcium intake, which will ultimately result in very low bone mineral density leaving an athlete with poor bone health and more prone to injury. On the other end of the spectrum, there are other events in track and field where greater total body mass, especially muscle mass, may be an advantage (e.g., shot put, hammer throw, etc.). Athletes in these events may resort to differ- ent methods to gain mass. Again, the addition of more total body mass in these athletes does not always guarantee success and sometimes can lead to imbalances in muscle composition leading to injury. The different competitive and health imperatives present a conflict for some track and field athletes, for whom eating disorders are exacerbated. Therefore, an important step in maintaining a track and field athlete's health and performance is the ability to accurately measure and assess an athlete's body composition. This article explores the various methods used to determine body composition, as well as the ranges in percent body fat that exist in track and field athletes. The assessment of an athlete's physique and body composition to gain a competitive advantage is not a new idea. On the contrary, the concept of analyzing an athlete's physique has been around since the ancient Greek Olympic Games. Some might say the Greeks were obsessed with an athlete's physique, often depicting athletes in statues and vases. The Renaissance period led to an era of advanced study in the dimensions of the human body. Individuals such Leonardo da Vinci, not only extensively painted and sculpted the human figure, but also made detailed measurement of the anatomical proportions. Today there are a number of tools at our disposal, not only to measure the physique of an athlete, but also the specific amounts of muscle, fat and bone (i.e., body composition). To a large degree, an athlete's body type or build is limited by what they have inherited from their parents. This does not imply that there is nothing that can be done to change or improve themselves. While body type or build can only be altered slightly, substantial changes can occur in body composition with diet and training. These changes in body composition can be of major importance to achieving optimal athletic performance. METHODS OF BODY COMPOSITION ANALYSIS The oldest method of evaluating an athlete is simply looking at the body size, or the height and mass, of the individual. The distinctions of being classified as short or tall, large or small, heavy or light, depend entirely on the sport. A height of 188 cm (74 in) would be tall for a distance runner, but the average height of a decathlete (Table 6). Similarly, a weight of 78.9 kg (174 pounds) would be an average weight for a sprinter, but too heavy for a distance runner (Table 4). Therefore, body size must be considered relative to the event that the athlete participates in. The use of body size is frequently used by doctors and insurance companies, etc. to gauge the health of an individual. By taking the weight of an individual in kilograms and dividing it by the height in meters square you can determine the body mass index (BMI) of an individual. In males, a BMI greater than 25 kg/ m2 is considered overweight. This method has limitations, for example if we look at decathletes we would find athletes who are considered overweight by this method. Obviously, the problem with using BMI in athletic populations that carry considerable amounts of muscle is they appear to be overweight using this simple system. This problem is even more evident in professional football players where almost the entire National Football League is considered overweight and a large number of players are considered obese (BMI >30 kg/ m2) (Dengel et al., 2014). Knowing only the weight of an athlete often provides a minimum of what an athlete or coach needs to know to develop an effective training program. Body composition analysis provides additional information to both the coach and the athlete. For example, to know that an athlete has a total weight of 200 pounds provides us with very little information of the quality of the 200 pounds. Body composition analysis could tell that coach that of those 200 pounds, 10 pounds are fat and the remaining 190 pounds are fat-free mass (i.e., muscle and bone mass). This provides both the coach and athlete with considerably more insight into the athlete's body. In this example only 5 percent of this athlete's body weight is fat, which is about as low as any male athlete should go (Table 1). This athlete would then realize that his body composition is ideal, and he should not try to lose weight, even though he may be overweight by the standard height-weight charts. This example shows the value of utilizing body composition analysis instead of just a scale. Currently, there are a number of methods and techniques for the estimation of body composition, ranging from simple field methods (i.e., skin fold measurements) to more advanced laboratory methods (i.e., dual energy X-Ray absorptiometry [DXA], underwater hydrostatic weighing). Before selecting a method, the coach or athlete should consider the availability of the necessary equipment, financial costs, safety precautions and accuracy of the method. Regardless of the cost or degree of accuracy it is important to remember that the measurement of body composition is not an exact science. All methods are prone to some degree of error. Therefore, it is important for the coach and athlete to think of the measurement of body composition as a range that is associated with the error of the measurement versus a precise value. This paper will explore the different methods of determining body composition and will give the values found in various track and field athletes. It is important to remember that the values reported in this paper are from elite and Olympic caliber athletes. Therefore, care should be used when comparing these values to high school or even college athletes. In addition, though we give a single value it is important to look at this value as the midpoint of a range. For example, if the percent body fat value for male sprinters is 9.1 percent, one should think that the range would be about 2 percent above and below this value. So male sprinters would have a range between 7.1 percent - 11.1 percent. In looking at Table 2, you can see that the two studies listed on male sprinters report percent body fat values of 8.4 percent and 9.8 percent. One important thing about under-standing body fat in the human body is that body fat really consists of two unique areas: one called essential body fat and the other termed storage fat. For health purposes, the body has a need for a certain level of body fat that is present in nerve tissues, bone marrow, and organs (all membranes). The other location of body fat in our body is the storage area. This represents an energy reserve that accumulates when excess energy is ingested and decreases when more energy is expended than consumed. In males, essential body fat is approximately 2-5 percent of total body mass for men and for women it is approximately 12-15 percent of total body mass (Table 1). Women have more essential body fat than men because of childbearing and hormonal functions. In general, the total body fat percentage (essential plus storage fat) is between 18 percent and 25 percent for men and between 25 percent and 31 percent for women (Table 1). Though percent body fat for athletic populations is listed between 6 percent and 13 percent in males and 16 percent and 20 percent for females, different sports have different body composition requirements. In some contact sports such as football, a higher body weight is sometimes seen as an advantage while in sports such as gymnastics, basketball and other weight-bearing activities, a lower body weight and high power-to-weight ratio are extremely important. Therefore, in these sports both low body fat and low body weight are necessary. Even in a sport like football there is a wide range of acceptable body fat percentages (Dengel et al., 2014). In football the desired body fat percentage was dependent to a large degree on the position of the player with wide receivers having significantly lower body fat percentages than lineman and even linebackers (Dengel et al., 2014). Track and field is much like football in that there is a wide range of body fat percentages in the athletes that participate. The body fat percentage in track and field athletes is dictated in a large degree to the events that the athlete participates in. It should be noted that there is no true accepted percentage body fat standard for the various events that make up track and field. An athlete's ideal body composition should be discussed on an individual basis with the coach, physiologist and nutritionist or dietician. In addition, body weight and body composition should be discussed in relation to functional capacity and exercise performance. Before discussing the body fat percentages of track and field athletes let's first examine the different methods of determining body composition. When examining the various method to estimate body composition it is important to remember that the body is actually made up of a variety of different types of tissues and fluids. Whatever type of method is used to estimate body composition it has to deal with these different types of tissues and fluids. The simplest, and typically the cheapest, methods to measure body com-position usually use what is referred to as two-component model to estimate body composition. In other words, body com-position methods that utilize a two-component model will group the total body mass into one of two components. The first component is the amount or mass of fat in the body and the second component is the amount of fat-free mass contained in the body. This second component (i.e., fat-free mass) places bone and muscle in the same component. Body composition methods based upon two-component models are associated with greater error in the measurement than methods based on multi-component models. The three-component models divides the fat-free mass component into bone mass component and a lean mass (i.e., muscle) component. Though bone does not typically account for a great amount of the total body mass of an individual the three-component model is more accurate than the two-component model. Unfortunately, the technology needed to measure three components accurately is expensive. Therefore, devices measuring three components often cost more than those devices that only measure two components. TWO-COMPONENT MODEL METHODS By far, the most common methods of estimating body composition fall into those methods that utilize a two-component model. The most often utilized two-component method of determining body composition is the measurement of skin fold thickness. (See skin fold photo) This relatively inexpensive field method uses a special caliper to make measures of skin fold thickness at a variety of sites around the body. These measures of subcutaneous fat are then inserted into an appropriate formula to calculate the body fat percentage. The formula chosen should be specific to the sex, age, and ethnicity of the individual. This method is dependent to some degree on the individual administering the test as well as the formula selected. The standard error of the skin fold technique is about 3-4 percent (Williams et al., 2013). Another common two-component method of measuring body composition is hydrostatic weighing, (See Hydrostatic Weighing Photo) which is also known as underwater weighing. This method is based upon Archimedes' principal that an object immersed in water is acted upon by a buoyancy of the object and the amount of fluid the object, in this case a body, displaces. Because fat is less dense than either bone or muscles it weighs less in water. In this method an individual is weighed and then is placed inside of tank of water. After blowing out all of the air in his or her lungs and submerging his or her head below the water, he or she is weighed again in the water. After correcting for water temperature and subtracting the amount of air retained in the lungs after a forced expiration (i.e., residual lung volume), body volume equals the loss of weight in water. This method requires the investment of a water tank and special weighing system that makes the initial costs of the system rather expensive. At one time this method was considered the gold standard for measuring body composition, considering that the standard error of this technique is approximately 2-2.5 percent (Williams et al. 2013). Another technique that uses the two-component model to estimate body composition is air displacement plethysmography. Similar to underwater weighing, however, instead of measuring the amount of water a subject displaces, this method uses air and calculates the volume of air that a subject displaces. This method requires a subject to sit in a sealed chamber designed to measure the amount of air they displace. It has a number of advantages over hydrostatic weighing such has not having to go underwater and it is simple to use. The system can be costly to purchase and it has a similar degree of standard error (2-3 percent) as hydrostatic weighing (Fosbol • Zerahn, 2015). Another popular field method of estimating body composition is bioelectrical impedance analysis, which is based on the principle of resistance to an electrical current that is applied to the body. The greater the water content of the body the less the resistance and ultimately the greater the body density. Just like the skin fold thickness technique, the resistance is put into an equation to calculate percent fat. This equation is typically programmed into the machine and is often based upon age, sex and ethnicity. Measurement of body composition by bioelectrical impedance can be affected by a number of factors such as: a previous bout of exercise, body position, skin temperature, dietary intake, and hydration status (Fosbol • Zerahn, 2015). The accuracy of bioelectrical impedance is similar to that of the skin fold technique (3-4 percent), but does not require the technical skill of a skin-fold user (Williams et al., 2013). (See BIA photo) THREE-COMPONENT MODEL METHODS As we mentioned, methods that estimate body composition using a three-component model are often more expensive and, until recently, mainly used in clinical setting. Recently, DXA (See DXA photo) has started to be used to estimate body composition in athletes and many professional and college teams have access to or own DXA devices. To determine the three components in the body, DXA uses two distinct low-energy X-Ray beams with short exposure and low radiation dosage to penetrate bone and soft tissue areas to a depth of approximately 30 cm. The subject lies supine on a table so the source and detector probes slowly pass across the body over a 12-minute period. Computer software reconstructs the attenuated X-Ray beams to produce an image of the underlying tissues and quantity bone mineral content, total body mass and lean tissue mass. The ability to measure bone density provides useful information regarding the thickness of bones, which is especially important for women and distance runners who may be at risk for osteoporosis. In addition, DXA may be used to assess regional fat deposit as well as abdominal visceral fat. The regional measurement of body composition allows one to compare legs versus arms and right versus left side body composition. The method can estimate body fat with an error of less than 2 percent (Shephard, 1991). MRI and computed tomography (CT) are also three component methods to determine body composition. However, both of these methods are limited by cost and/or radiation, which makes their use limited in the determination of body composition. These two methods of measuring body composition are expensive and in the case of CT involve higher levels of radiation. Typically, these two methods are only used in clinical or research settings. ANALYSIS OF BODY COMPOSITION IN TRACK AND FIELD ATHLETES So let's look at the body composition of various track and field athletes. It is important to remember that the athletes we are going to examine are elite and Olympic caliber athletes and therefore, one should be mindful in applying these values to high school and collegiate athletes. Table 2 lists the height, weight and body fat percentages of male and female sprinters from junior to Olympic caliber athletes. On average, males sprinters are 177.5 cm tall (69.9 inches) and weigh on average 69.5 kg (153.2 pounds). However, it is important to remember that this is the average and there are exceptions. Usain Bolt, for example, is 195.6 cm (77 in) tall and weighs 85.7 kg (189 pounds). For male sprinters, the average percent fat is 9.1 percent. Female sprinters are 167.4 cm tall (65.9 in), weigh, on average, 57.0 kg (125.7 pounds), and have an average percent body fat of 13.7 percent. Table 2 demonstrates the wide range of body fat percentages from 10.7 percent for junior national women who may have not fully reached sexual maturation yet to 19.4 per-cent for collegiate women who may not be at the same level as Olympic female sprinters. This does not mean that all female sprinters need to be 13.7 percent to make the Olympics it just demonstrates the range of percent body fat that can exist in this population. Today's middle distance runners typically require a unique blend of talents, requiring a high aerobic capacity like a distance runner, but also a well-developed anaerobic system like a sprinter. The body composition of these athletes is similar to that of their sprinter teammates. On average male middle distance runners are 179.8 cm (70.8 inches) tall, weigh 67.2 kg (148.1 pounds) and have an average percent body fat of 9.3 percent. Female middle distance runners are 165.1 cm (65.0 in) tall, weigh on average 52.9 kg (116.6 pounds) and an average percent body fat that is similar to female sprinters 14.1 percent. Again, Table 2 shows us the wide range of percent body fat in these athletes from 19.1 percent body fat in collegiate middle distance runners to 10.9 percent body fat in Olympic female middle distance runners. Long distance runners are often smaller than sprinters or middle distance runners, with male distance runners being 176.4 cm (69.4 inches) tall, weigh 64.4 kg (141.0 pounds) and on average have a percent body fat of 8.0 percent. Females also tend to be smaller than their female sprinter counterparts being 161.6 cm (63.6 inches) tall and weight 48.1 kg (106.0 pounds). Surprisingly, they have a little more body fat (15.9 percent) than female sprinters. Male marathoners were 173.1 cm (68.1 in) tall, weighed 61.0 kg (134.5 pounds), and had an average percent body fat of 6.9 percent. Female marathoners were 164.7 cm (64.8 in) tall and weighed 57.0 kg (125.7 pounds). In regards to percent body fat, female marathoners were similar to female long distance runners at 16.3 percent. A racing walker, which is only a male Olympic sport, is similar in height (173.2 cm, 68.2 inches) and weight (62.9 kg, 134.3 pounds) to male marathoners. They did tend to have a little more body fat at 9.4 percent than male marathoners, but are still very lean.
Individuals who compete in the shot put, discus, javelin or hammer are often grouped together as throwers. This may be a little deceiving since an individual competing in the javelin will have a body type that is often much different than the athlete who competes in the shot put. Male throwers are 186.6 cm (73.5 inches) tall and weight 105.4 kg (232.4 pounds). Female throwers are 170.8 cm (67.2 inches) tall and weight 76.2 kg (168 pounds). On average, male throwers had a body fat of 15.9 percent, while females had a percent body fat of 25.4 percent. It is important to remember, these data are mostly from the 1970s and 1980s. Both male and female throwers have gotten a lot taller and weigh a lot more today. So the weight and height of throwers today is probably much greater than what was reported in these studies, however the percent fat values may actually be similar to what was reported in these studies. Table 6 contains body fat information on jumpers (i.e., high jump, long jump, and triple jump) as well as information on pole-vaulters, decathletes, and pentathletesheptathletes. Male jumpers are 182.8 cm (72.0 inches) tall and weigh 73.2 kg (161.4 pounds). Male jumpers on average had a percent fat of 8.9 percent. Not too surprising, this amount of body fat was almost identical to the 9.1 percent body fat that sprinters had. Female jumpers are 169.7 cm (66.8 inches) tall and weigh 56.4 kg (124.3 pounds), generally having a percent body fat of 12.4 percent, which is identical to the level found in the two studies that examined female Olympic sprinters. Male pole-vaulters had a similar level of percent fat at 8.8 percent as jumpers and sprinters and were 179.7 cm (70.7 in) tall and weighed 70.6 kg (155.6 pounds). Though to date there are no studies on percent fat levels in female pole-vaulters, given that male jumpers, sprinters and pole vaulters are very similar in body composition it is reasonable to assume that female pole vaulters will have similar body fat levels as their jumping and sprinting counterparts. The multi-event athletes (i.e., decathletes, pentathletesheptathletes) often require a unique blend of strength, speed and endurance. Therefore, it is not surprising to see that they have a little more body fat (10.3 percent in decathletes and 13.7 percent in pentathletes heptathletes) than jumpers and sprinters. Male decathletes were 181.3 cm (71.4 inches) tall and weighed 77.5 kg (170.8 pounds). Female pentathletesheptathletes were 172.3 cm (67.8 inches) tall and weighed 63.6 kg (140.2 pounds). As with throwers, there has, in all likelihood, been an increase in the size (i.e., height, weight) of both decathletes and pentathletesheptathletes since these original studies were done. CONCLUSION In summary, while an athlete's body composition may help to optimize their performance in a given sport, it is important to remember that the purpose of this article is to act as a guide concerning the method of acquiring data on body composition and utilizing this data to alter training techniques in track and field athletes. It is our hope that this article will provide coaches and athletes with a reference, but in the end, it is important to remember that track and field athletes come in a variety of shapes and sizes and, ultimately, body compositions. The information provided in this article should not take the place of medical advice. Any specific questions should be directed toward appropriate health care provides (medical doctors, pharmacists, registered dieticians, etc.). REFRENCES Boileau, R.A., Mayhew, J.L., Riner, W.F., • Lussier, L. (1982). Physiological characteristics of elite middle and long distance runner. Canadian Journal of Applied Sport Sciences 7(3):167-172. Christensen, C.L., • Ruhling, R.O. (1983). Physical characteristics of novice and experienced woman marathon runners. British Journal of Sports Medicine 17(3):166-171. Costill, D.L., Bowers, R., • Kammer, W.F. (1970). Skinfold estimates of body fat among marathon runners Medicine and Science in Sports 2: 93-95. Dengel, D.R., Bosch, T.A., Burruss, T.P., Fielding, KA., Engel, B.E., Weir, N.L., • Weston, T.D. (2014). Body composition of National Football League players. Journal of Strength and Conditioning Research 28(1):1-6. deGaray, A.L., Levine, L., Carter, J.E.L. (Eds.). (1974). Genetic and anthropological studies of Olympic athletes. New York: Academic Press. Fahey, T.D., Akka, L., • Rolph„ R. (1975). Body composition and VO2 max of exceptional weight-trained athletes. Journal of Applied Physiology 39:559-561. Fosbol, M.O., • Zerahn, B. (2015). Contemporary methods of body composition measurement. Clinical Physiology and Functional Imaging 35:81-97. Graves, J.E., Pollock, ML., • Sparling, P.B. (1987). Body composition of elite female distance runners. International Journal of Sports Medicine 8:96-102. Houtkooper, L.B., Mullins, V.A., Going, S.B., Brown, C.H., • Lohman, T.G. (2001). Body composition profiles of elite American heptatheletes. International Journal of Sport Nutrition and Exercise Metabolism 11:162-173. Malina, R.M., Harper, A.B., Avent, H.H., • Campbell, D.E. (1971). Physique of female track and field athletes, Medicine and Science in Sports and Exercise 3: 32-38. Pollock, M L., Gettman, L. R., Jackson, A., Ayres, J., Ward, A., • Linnerud, A.C. (1977). Body composition of elite class distance runners. Annals New York Academy of Sciences. 301:361-370. Rusko, H., Havu, M., • Karvinen, E. (1978). Aerobic performance capacity in athletes. European Journal of Applied Physiology 38:151-159. Shephard, R.I. (1991). Body composition in biological anthropology. Cambridge: Cambridge University Press. Tanner, J.M. (1964). The physique of the Olympic athlete. London: George Allen and Unwin. Thorland W.G., Johnson, G.O., Fagot, T.G., Tharp, G.D., • Hammer, R.W. (1981). Body composition and somatotype characteristics of junior Olympic athletes Medicine and Science in Sports and Exercise 332-338. Wilmore, J.H., Brown, CH., • Davis, J.A. (1974). Body physique and composition of the female distance runner. Annals New York Academy of Sciences 301:764-776. Williams, M.H., Anderson, D.E., •bull; Rawson, E.S. (2013). Nutrition for Health, Fitness and Sport (10th ed.) McGraw Hill. 0 Donald R. Dengel, Ph.D., is a Professor in the School of Kinesiology and Director of the Human Performance Core and Densitometry Services in Clinical and Translational Science Institute at the University of Minnesota. Dr. Dengel may be reached at denge001@umn.edu. Olivia Dengel is a cross country and track and field athlete at the College of Saint Benedict majoring in biology. |
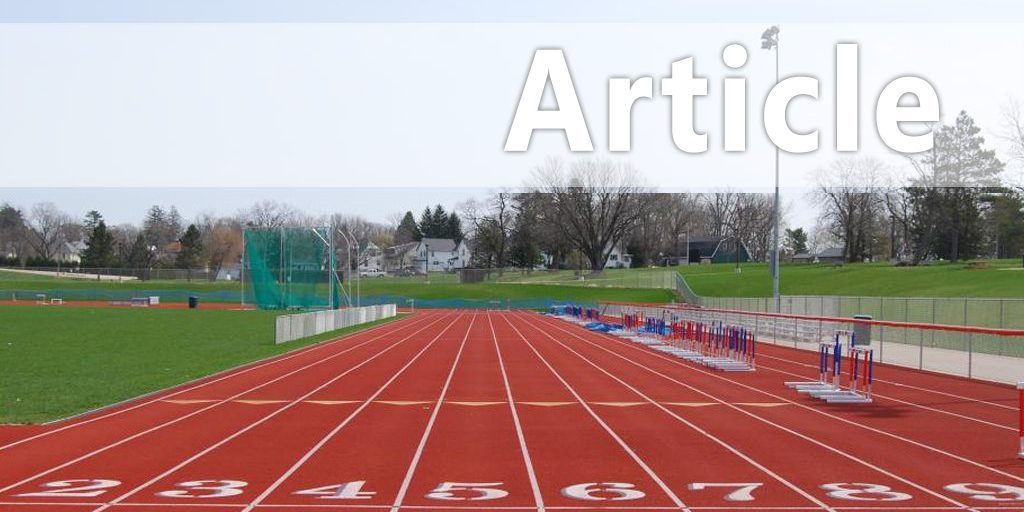